Making Flies Forget
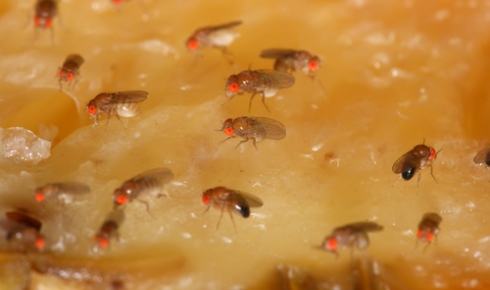
A surprising number of pathways associated with memory, learning and behaviour are conserved across humans and flies. James Higham looks at how modelling dementia in Drosophila is helping the development of more precisely targeted drugs
The Biologist 64(6) p22-25
Change is needed in Alzheimer's disease (AD) research. Despite substantial work in this field, current treatments do not target the underlying cause of disease, and are limited in number and effect. To add to the problem, drugs in AD clinical trials have a gloomy failure rate of 99.6%[1].
Given the immense personal, social and economic burden of AD – which is only set to increase as the population ages – new treatments are desperately needed, and a greater understanding of the disease process is required. Could the fruit fly (Drosophila melanogaster) be the key to learning more about the cellular and molecular underpinnings of AD?
Drosophila has been used in scientific research for over a century and, in recent decades, has become widely used in neuroscience. Flies' short lifespan (60 days) allows the rapid study of age-related processes. They are relatively inexpensive and easy to maintain. There also exist well-characterised techniques for manipulating gene expression in flies[2].
Gene expression levels can be elevated or suppressed, or a mutation included, in a cell-specific manner. This means that the expression of a gene could be blocked in only the neurons involved in memory formation, allowing the study of this gene in memory without contaminating the effect by having the gene manipulation present in other cells. Importantly, flies demonstrate key behaviours that are also found in humans – such as learning, memory, sleep/wake cycles and aggression – which appear to have conserved mechanisms. Therefore, disease-related changes in behaviour identified in humans can be studied in the fly and researchers can go on to examine the causative mechanisms at the cellular and molecular level.
In humans, mutations in the CASK gene lead to profound intellectual disability[3]. Drosophila that lack the CASK gene are unable to form memories[4] showing that these genes have similar functions in humans and flies. When researchers took flies lacking CASK and inserted a normal copy of the human CASK gene, they formed memories just as well as wild-type flies[4].In other words, a human gene product is able to function in the memory-forming pathway of a fly brain. At the very least, this illustrates the molecular similarities between us and flies (think before you swat!).
So, flies represent a high-throughput model for understanding disease processes and finding potential new treatments. How have they helped in AD research and how may they be used in the future?
For flies to be of use in this area, they must exhibit similar defects to humans with AD. Human AD is characterised microscopically by the presence of misfolded protein aggregates, which are toxic to the surrounding brain tissue[5]. Engineering flies to express the human genes coding for these proteins causes neurodegeneration[6], memory impairment[6] and disrupted sleep/wake cycles[7], all of which are seen in humans with AD.
Work on sleep/wake disturbances, in particular, has highlighted how flies can be useful in understanding pathophysiology. In one Drosophila model of AD[7], the affected flies sleep less at night and exhibit restlessness, as seen in the human form of the disease. Depriving the flies of sleep worsened the accumulation of toxic protein, while artificially augmenting sleep alleviated accumulation somewhat.
By placing tiny electrodes into the flies' brains and measuring neuronal electrical activity, researchers found AD flies have elevated activity in cells controlling sleep/wake activity. Sleep-deprived flies (both with and without the AD protein) had a greater elevation of cellular activity. This increased activity was attributed to the downregulation of processes that normally suppress electrical activity.
Blocking this increased cellular activity reduced protein aggregation in much the same way as augmented sleep. These results demonstrate a complex interaction between the toxic AD protein, sleep and the regulation of cellular electrical activity.
We can take advantage of this interaction to start to design treatments: could blocking increased electrical activity and extending sleep times reduce the burden and progression of disease? It has already been shown that artificially increasing sleep can restore long-term memory in a similar fly model of AD[8]. What's more, drugs known to suppress neuronal activity prolonged the lifespan of AD-affected flies[7]. This indicates that perturbed neuronal function impacts disease trajectory as well as behaviour, and restoring normal function delays disease progression.
While it is important to remember that these models only consider one component of AD, they still present promising avenues that warrant further investigation.
These examples alone show how using flies can elucidate the mechanisms underlying behavioural changes in AD. However, flies are also excellent screening tools for potential treatments. Perhaps flies could be the first rung on the screening ladder, being used for the identification of drug targets that can then be passed on for validation in mammalian model organisms and, eventually, for safety and efficacy testing in humans.
Drosophila would be key for the high-throughput identification and testing of targets with relatively low cost and resource use. This would require active collaboration between Drosophila, mammal and clinical researchers, which could be aided by the formation of drug discovery units within and between universities and hospitals.
Such units would enable data and resource sharing, and are likely to focus on esoteric targets that may not be of interest to pharmaceutical companies, but are important nonetheless. In terms of drug design, pharmaceutical companies have often manufactured many compounds with subtle changes and tested them on the target, which is time- and resource-consuming.
However, computer modelling of drug-target interactions (to find the most effective drug structure, which can then be tested in animal models), favoured by universities, could be more effective. Prioritisation of the most promising targets from an early stage would enable resources to be used more productively, and is logistically far simpler when data is shared and projects are managed collaboratively.
Drosophila has contributed enormously to the understanding of AD, among numerous other diseases. It will continue to be at the forefront of research and, if utilised efficiently as part of a collaborative effort with other research areas, can become an even more powerful model organism.
Aversive olfactory conditioning
A group of flies is exposed to a particular odour with an aversive stimulus (such as a small electric shock) and then a second odour with no electric shock. After a rest time corresponding to the type of memory being tested (short term: two minutes; long term: 24 hours), flies are presented with both odours in a T-maze. If a fly has associated an odour with an aversive stimulus, it will avoid it.
Locomotor activity monitoring
Single male flies are placed into small tubes with food and the tubes are loaded into monitors that project infrared beams across the middle to count the number of times the beam is crossed by the fly in a given time period. This can be used to monitor activity and sleep throughout the day and night. The integrity of flies' circadian clocks can be tested by entraining them to a light/dark cycle and then changing to permanent darkness, and monitoring if activity continues to peak at dawn and dusk.
James Higham AMRSB is an honorary research fellow at the University of Bristol's School of Physiology, Pharmacology and Neuroscience.
References
1) Cummings, J. L. et al. Alzheimer's disease drug-development pipeline: few candidates, frequent failures. Alzheimers Res. Ther. 6(4), 37 (2014).
2) Jones, W. D. The expanding reach of the GAL4/UAS system into the behavioural neurobiology of Drosophila. BMB Rep. 42(11), 705–712 (2009).
3) Hackett, A. et al. CASK mutations are frequent in males and cause X-linked nystagmus and variable XLMR phenotypes. Eur. J. Hum. Genet. 18(5), 544–552 (2010).
4) Malik, B. R. et al. CASK and CaMKII function in the mushroom body neurons during Drosophila memory formation. Front. Neural Circuits 7, 52 (2013).
5) Armstrong, R. A. Plaques and tangles and the pathogenesis of Alzheimer's disease. Folia Neuropathol. 44(1), 1–11 (2006).
6) Iijima, K. et al. Dissecting the pathological effects of human Abeta40 and Abeta42 in Drosophila: a potential model for Alzheimer's disease. Proc. Natl. Acad. Sci. USA, 101(17), 6623–6628 (2004).
7) Tabuchi, M. et al. Sleep interacts with abeta to modulate intrinsic neuronal excitability. Curr. Biol. 25(6), 702–712 (2015).
8) Dissel, S. et al. Enhanced sleep reverses memory deficits and underlying pathology in Drosophila models of Alzheimer's disease. Neurobiology of Sleep and Circadian Rhythms 2, 15–26 (2017).