Viruses That Kill Cancer
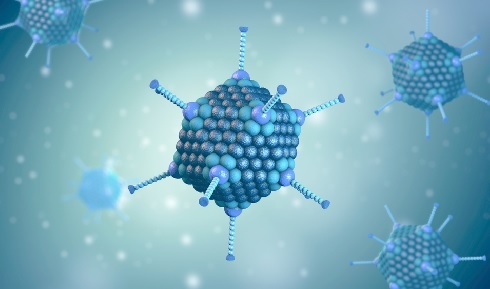
Researchers are getting closer to producing oncolytic viruses that can selectively infect and kill malignant cells, leaving healthy cells unaffected
Feb 22nd 2021
For many years the word virus has been associated with infections, disease and death. However, there are numerous viruses that either exhibit a natural preference for destroying cancer cells or can be genetically modified to target cancer cells selectively. These are known as oncolytic viruses (OVs).
At the beginning of the 20th century it was observed that some patients with cancer who then had a viral infection showed a decrease in their tumour size[1]. This led to the development of a range of theories and ideas exploring the relationship between viruses and cancer.
By the 1950s, in a series of experiments, patients with cancer were given infectious body fluids or infected tissue harvested from other patients with ongoing virus infections. The viruses used included hepatitis, influenza and picornavirus. Tumour remission was observed, yet it would only last one to two months, and often the accompanying illness and morbidity as a result of the infection of normal tissues was unacceptable[2]. From 1950 to 1980 no positive clinical outcomes were achieved as scientists struggled to control the virulence[3,4]. Interest faded and researchers largely abandoned the idea.
However, genetic engineering technologies developed in the 1990s rekindled interest in OVs. These new technologies enable scientists to alter viruses, inserting or deleting genetic material to make them more specific to tumour cells and less dangerous to healthy cells.
Targeting abnormal cells
Both natural and engineered oncolytic viruses take advantage of the many genetic differences between cancer cells and normal cells. Some, for example, infect cancerous cells more readily simply because their viral defences are defective. The overexpression of certain surface receptors may further help OVs to bind to and infect malignant cells. The abnormal activation of genes involved in cell growth and proliferation, inactivation of genes involved in apoptosis and DNA repair, abnormal growth signals and the hypoxic environment found in tumours can also help these viruses replicate aggressively in cancer cells[3].
For example, the human reovirus is a naturally occurring OV that targets cancer cells that have an activated Ras pathway. Ras-regulated signal pathways control cell proliferation, cell adhesion, cell migration and apoptosis, and tend to be deregulated in cancer cells. Studies have shown that Ras-defective cells have compromised antiviral activity compared with untransformed cells to the extent that reovirus can infect them[6]. Similarly, a modified adenovirus, Onyx-015, is able to replicate only in cells lacking a tumour-suppressing protein known as p53. The unmodified virus normally replicates in human cells thanks to a gene within its genome that inactivates p53; when this gene is removed from the virus it can then only replicate in cells where the p53 gene is defective – i.e. cancer cells.
Once OVs have infected and multiplied within a malignant cell the cell bursts (cell lysis). The release of the cell’s contents – including viral particles and tumour antigens – into the surrounding tissue then triggers waves of local immune system activity. Not only are the cancer cells being infected and killed, but the resulting stimulation of the immune response gives rise to an augmentation of the body’s anti-tumour immunity.
Immune cells such as T-cells and macrophages start clearing the tumour cells, as well as the OV itself, which can lead to systemic and sustained anti-tumour immunity. Intravenous injection of OVs, in high enough doses that the body’s immune system cannot quickly clear them all, could help eliminate secondary tumours and metastatic cancer cells that have travelled throughout the body[5], one of the great remaining challenges of cancer therapy.
Binding to tumours
Recent advances in molecular biology enable researchers to create viruses that bind to entry receptors highly expressed on tumours, and for safety can only replicate in cancer cells. They can also insert genes that will induce the infected cell to produce therapeutic or immune-stimulating compounds. For example, OVs can also be used as vectors to deliver ‘suicide genes’, also known as prodrug transforming genes. These encode proteins that help to metabolise a separately administered non-toxic and inactive drug into a cytotoxin (active drug), in effect making a compound toxic only to those cells infected with the virus[4].
Another example of combined therapy combines OVs with ionising radiation. The viruses can be used as radiosensitisers, making tumour cells much more sensitive to radiation than healthy cells. OVs have also been engineered to encode transmembrane proteins responsible for the uptake of iodide. This way, radioactive iodine can be actively transported into the infected cancer cells, helping both localised radiotherapy as well as tumour imaging[5,6,7].
As with any cancer therapy, there are downsides to this approach. The immune system becomes activated soon after the virus infects cells and viruses can be cleared from the bloodstream quickly before the effects are realised. Another problem is pre-existing immunity[2]. Most of the population get vaccinated against viruses or encounter them in the course of their life, resulting in an immunological memory. The immune system may quickly recognise a virus a person has been exposed to or has been vaccinated against, even if it has been modified. Therefore the host’s immune system might neutralise the virus before it can have any effect.
Combination therapies
It is believed that the most effective use of OVs will be when they are co-administered with other therapies. To obtain strong, long-lasting results and complete tumour disappearance, OVs could be combined with other immunotherapies such as checkpoint inhibitor drugs or with radiotherapy. In addition, a better understanding of the real-time progression of the virus in the body is vital to the timing of administration of other therapies in combination with the OV or right after viral clearance[3,8].
Currently, at least 50 trials of oncolytic viruses are being conducted at research institutions around the world. So far, adenoviruses, herpes viruses, measles viruses, coxsackie viruses, polioviruses, reoviruses, poxviruses, Newcastle disease viruses and others have been explored in pre-clinical or clinical trials for cancer therapy. To date, only one OV has been approved, by the Food and Drug Administration in the US: T-VEC, a modified herpes simplex virus approved for patients with melanoma in 2015[8]. The virus is injected directly into melanoma lesions, and in addition to directly killing cells, the virus also appears to trigger a heightened immune response to the tumour through the release of antigens shed from the infected cells and the release of the immune-stimulatory protein GM-CSF.
Scientific and technological advances, together with the knowledge development on genetic engineering and immunotherapy, have made it possible to improve new techniques for modifying viruses. However, further research should focus on combining the OVs with other immunotherapies as well as avoiding antibodies from pre-existing immunity in a patient. This could help to achieve the desired goal: to destroy tumour cells while causing no damage to healthy cells and tissues.
Belen Vilanova AMRSB is a master’s student at the University of Glasgow studying precision cancer medicine following a degree in biomedical science at Edinburgh Napier University.
1) Dock, G. The influence of complicating diseases upon leukaemia. Am. J. Med. Sci. 127(4), 563 (1904).
2) Kelly, E. & Russell, S. J. History of oncolytic viruses: genesis to genetic engineering. Mol. Ther.
3) Howells, A. et al. Oncolytic viruses – interaction of virus and tumor cells in the battle to eliminate cancer. Front. Oncol. 7, 195 (2017).
4) Zarogoulidis, P. et al. Suicide gene therapy for cancer – current strategies. J. Genet. Syndr. Gene Ther. 4 (2013).
5) Advani, S. J. et al. Increased oncolytic efficacy for high-grade gliomas by optimal integration of ionizing radiation into the replicative cycle of HSV-1. Gene Ther. 18(11), 1098–1102 (2011).
6) Martin, N. T. & Bell, J. C. Oncolytic Virus combination therapy: killing one bird with two stones. Mol. Ther. 26(6), 1414–1422 (2018).
7) Huber, S. M. et al. Ionizing radiation, ion transports, and radioresistance of cancer cells. Front. Physiol. 4 (2013).
8) Zhang, Q. & Liu, F. Advances and potential pitfalls of oncolytic viruses expressing immunomodulatory transgene therapy for malignant gliomas. Cell Death Dis. (6), 485 (2020).