The Silk Route
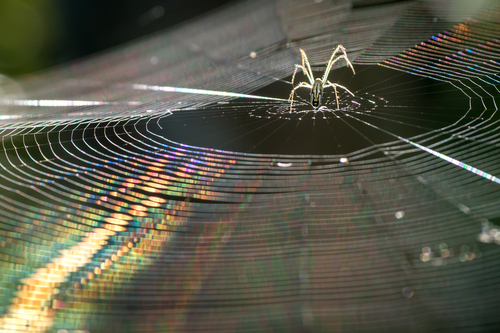
Spider silk is known for its remarkable strength but, as David Butler reveals, it has great potential for a range of medical uses
The Biologist Vol 61(5) p12-15
Solutions to problems in science and engineering are often found in structures, organisms and symbiotic relationships already seen in nature. Examples of this include the construction of artificial wetlands to reduce the effect of hazardous chemicals in agricultural runoff; the strategic use of trees in cities and other urban areas to filter harmful pollutants (The Biologist Vol 60 (1) p28); and the construction of high-strength materials based on the structure of the toco toucan (Ramphastos toco) beak (Seki et al., 2005).
So imagine a material that has properties covering a wide range of medical, biomedical and engineering applications. It is stronger and lighter than any manmade material. And it can be found in dusty cupboards, unkempt attics and in many areas of the natural world. In fact, it can be found wherever spiders live, as this incredible material is their silk.
Spider silks and their proteins have extraordinary properties. They can possess a tensile strength on a par with Kevlar, yet retain a low density, extraordinary ductility and elasticity. The silks are largely non-toxic to human cells and have relatively low immunogenicity (the likelihood that it will provoke an immune response).
The idea of using spider silk instead of traditional silkworm silk was explored during the last century, but spiders are territorial creatures and their aggressive nature meant spider farms never succeeded. However, thanks to recombinant DNA technologies, foreign protein expression and ongoing research, it is now possible to obtain artificial spider silk proteins and silk threads of the same molecular structure and integrity as natural ones.
Silk proteins
Spider silk threads are composed of various silk proteins called spidroins. Spidroins are very large proteins, with one of the largest having a molecular weight of over 300 kDa (300,000g per mole).
Spidroins are defined by the individual spider and the gland they are secreted from. Different arrangements of spidroins give rise to the variability of spider silk, and there are seven silk types in total (Hinman et al., 2000). The four well-studied spidroins are from the golden silk orb-weaver (Nephila clavipes) and are the Minor Ampullate Spidroin I (MiSpI), Minor Ampullate Spidroin II (MiSpII), Major Ampullate Spidroin I (MaSpI) and Major Ampullate Spidroin II (MaSpII).
The major and minor spidroins are similar in structure, although the minor ones have less tensile strength compared with the majors and have little or no elasticity (Hinman et al., 2000). It is for these reasons that the major spidroins have been the focus of more research.
As with all proteins, spidroins are made up of sequences of amino acids. Alanine, glycine, leucine, proline, tyrosine and phenylalanine feature heavily in these sequences, although other amino acids are present in trace amounts. Small sequences of amino acids are repeated in so-called 'peptide motifs', which give rise to the secondary and tertiary structures of the resulting proteins (see box).
There is also some level of hydrophobic and hydrophilic interaction within the spidroin molecules. Polyalanine regions within the protein molecule are hydrophobic, and provide the overall silk structure with extreme tensile strength by linking with other hydrophobic polyalanine regions in nearby protein structures to form very strong bonds. The hydrophilic regions are characterised by an abundance of glycine, and give elasticity to the silk thread fibre (Xia et al., 2012).
Research on the properties of silk from various families of spiders has determined that the silk and spidroins from the spider Nephila clavipes has the most promising characteristics. The tensile strength of this silk has been reported to be far greater than steel and, in some cases, that of Kevlar weight for weight. It is because of these fantastic properties that Nephila clavipes is the silk that is most commonly used in recombinant technology.
Spidroin motifs
The common motifs or repeated amino acid sequences in the major spidroins are GPGXX, GA and GGX (G is glycine, P is proline and A is alanine, while X represents other, non-repeating amino acids). It is thought that repeated complexes of GPGXX and GGX can form spring-like structures, giving the protein elasticity. Non-repetitive 'spacer regions' are thought to cross-link regions of the entire silk fibre.
Different silks are produced for each different use:
- Web frame
- Core fibres of adhesive spiral
- Joining fibres
- Web reinforcement
- Cocoons
- Swathing and egg sack
Foreign expression and the E. coli workhorse
A wide range of host organisms can be used to express spidroin proteins, including bacteria, transgenic animals and also some plants, each with their own benefits. However, with the introduction of foreign genetic information, there is always the problem of the host's capability to express the protein properly, especially proteins over a certain size.
Using E. coli to express spider silk has shown very promising results, although initially there were multiple problems with the organism as a vector (Xia et al., 2012). The actual genetic code for the spidroins is so large and rich in guanine and cytosine that E. coli's expression systems could not process it, and the resulting spidroins were of low molecular weight and malformed. Also spidroins contain certain amino acids that E. coli doesn't metabolise readily, further inhibiting their successful expression.
However, in 2012, a team of researchers in Korea overcame these complications to successfully express a large, 285 kDa spidroin based upon the Nephila clavipes MaSpII (Xia et al., 2012). Using metabolic engineering, construction of genetic code to express an abundance of the protein motifs found in MaSpII, and overexpression of the precursors of the amino acids not natural to the E. coli metabolism, the expression was successful. Spinning of these spidroins into a thread resulted in a material with a tensile strength of 21 106 N/m2. This was seen as an absolutely remarkable breakthrough in terms of spidroin production and has led to great strides in development.
Expression of recombinant spidroins in mammalian milk has been in development for the past decade. The use of transgenic goats has been particularly successful: spidroins in the region of 140 kDa have been reported (Chung et al., 2012). As with the natural passage of genetics from parent to offspring, it is difficult to express the gene in all modified animals and only a fraction of modified animals pass the gene onto their offspring.
Transgenic plants have also been used in the production of recombinant spidroins. Transgenic tobacco and potato plants have enabled researchers to harvest large quantities of spidroins at low cost. Researchers successfully expressed spidroins of 100 kDa in tobacco and potato plants, at a concentration of 0.5% of the total cellular protein (Scheller et al., 2001).
Medicinal benefits
Both recombinant and natural spidroins have a variety of properties that make them useful in a range of potential medical applications. They biodegrade, they are stable over a large pH range, they have antimicrobial properties and they are relatively non-toxic.
To date, research has included the use of recombinant spidroins as wound dressings and gene delivery systems. There is also interest for expansion of research and technological development to include spidroins in cancer therapies, biomedical materials, polymers and structures, and as a textile material.
The antimicrobial properties of spidroins can be attributed to engineering antimicrobial peptides into the spidroin structure. One research study generated recombinant spidroins containing either human neutrophil defensin 2 (HNP-2), human neutrophil defensin 4 (HNP-4) or hepcidin (Gomes et al., 2011). Spidroins containing HNP-2 or hepcidin were assayed for antimicrobial activity against E. coli and Staphylococcus aureus. Antimicrobial activity was observed among varying concentrations of each spidroin form against the bacteria, but the spidroin that exhibited the most antimicrobial capabilities was the hepcidin-containing spidroin at concentration of 10 µgmL-1 against E. coli.
Experiments using spidroins as a wound dressing material have been done before on Sprague Dawley rats (Baoyong et al., 2009). After inflicting the rats with burn wounds from 90°C water, the population was divided into three groups: one received treatment with spidroin membranes, another was given collagen and the third had no treatment for their injuries. The results indicated that spidroin membranes stimulated faster wound healing and regeneration than in the negative control group and similar to the collagen group.
Using recombinant spidroins as a delivery method for genetic information has been investigated using spidroins containing tumour-homing peptides (THPs) biocomplexed with plasmid DNA (pDNA) encoding firefly luciferase (Numata et al., 2011). The biocomplex of spidroin, THP and pDNA was targeted towards MDA- MB-231 metastatic breast tumour cells in mice. The spidroins were expressed in E. coli, the tried and tested workhorse.
The spidroin pDNA complex delivered the luciferase into the tumour cells. The genetic information for firefly luciferase was up taken into the tumours and imaging of the mice demonstrated the expression of the luciferase gene. The investigation concluded that biocomplexed spidroins targeted for tumour cells exhibited specificity and also demonstrated that silk-based delivery system of genetic information is a feasible method of gene therapy treatment.
A positive future
The area of recombinant spider silk technologies is vast, with extraordinary research and development having been done in years past, and yet more waiting to be carried out in the years to come. Hopefully, within the next decade, the biomedical and commercial abilities of spider silk will be fully realised and utilised.
Hopefully, this fantastic technology may be used to alleviate problems such as post operation infection reduction, more efficient treatment for burn victims, and reduction in the environmental impact from discarded medical supplies. The list and possibilities are endless. It nearly boggles the mind.
David Butler AMSB recently graduated from the Waterford Institute of Technology in Ireland and is currently gaining work experience before deciding upon a suitable biotechnological postgraduate route.
References
Baoyong, L. et al. Evaluation of a new type of wound dressing made from recombinant spider silk protein using rat models. Burns 36(6), 891-896 (2009).
Chung, H et al. Recent advances in production of recombinant spider silk proteins. Current Opinion in Biotechnology 23(6), 957-964 (2012).
Eisoldt, L. et al. Decoding the secrets of spider silk. Materials Today 14(3), 80-86 (2011).
Gomes, S. C. et al. Antimicrobial functionalized genetically engineered spider silk. Biomaterials 32(18), 4255-4266 (2011).
Hinman, M. B. et al. Synthetic spider silk: a modular fiber. Trends in Biotechnology 18(9), 374-379 (2000).
Lee, K. S. et al. Molecular cloning and characterisation of the partial major ampullate silk protein gene from the spider Araneus ventricosus. Jnl of Asia-Pacific Entomology 15(4), 641-646 (2012).
Lefèvre, T. et al. Diversity of molecular transformation involved in the formation of spider silk. Jnl of Molecular Biology 405(1), 238-253 (2011).
Numata, K. et al. Spider silk-based gene carriers for tumour cell-specific delivery. Bioconjugated Chemistry 22(8), 1605-1610 (2011).
Scheller, J. et al. Production of spider silk proteins in tobacco and potato. Nature Biotechnology 19(6), 573-577 (2001).
Seki, Y. et al. Structure and mechanical behaviour of a toucan beak. Acta Materialia 53(20), 5281-5296 (2005).
Xia, X. et al. Native-sized recombinant spider silk protein produced in metabolically engineered Escherichia coli results in a strong fiber. PNAS 107(32), 14059-14063 (2012).