The value of venom
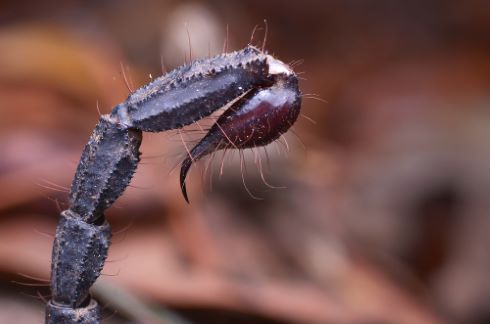
Venoms are a hugely unexplored group of complex bioactive compounds that do far more than just help animals kill their prey, writes Professor Sakthivel Vaiyapuri FRSB
20th February 2023
Venom is generally synonymous with death and misfortune, but there’s more to venom than you might think. Containing a mix of hundreds of proteins, peptides and other components that have evolved to disrupt normal physiological processes within a target organism or prey, venom’s primary function is to disable prey to facilitate feeding. However, this cocktail of powerful bioactive compounds, secreted from specialised glands and delivered through a wound, can have a range of biological functions, and venoms or venom-inspired compounds can have useful medicinal properties.
Venomous organisms differ from poisonous animals. Put simply, if something bites you and you die, then it is venomous; if you eat it and you die, then it is poisonous. While this is an oversimplified explanation, it does imply a key difference – that venom is delivered through specialised delivery systems (ie fangs) rather than ingested. Venom may not always be delivered in the form of a bite – spitting cobras can spit venom into the eyes – but mostly it will be transferred through a puncture wound.
If venom has evolved to facilitate feeding and predation, then does that mean venomous organisms are trying to feed on us? No. Humans are not a perceived food source for any venomous organism. Humans and venomous animals’ interactions, while they can be fatal, are an unfortunate side-effect of the evolution of their venom to target specific physiological processes in their prey that share homology with our own.
As our population continues to increase in number and expand into rural areas, human interactions with venomous animals are becoming more common. This forced cohabitation results in an increased number of what we call ‘envenomations’.

Convergent evolution
Venom is one of the most convergent traits known in evolution. It has evolved independently more than a hundred times, and is found in more than 200,000 species, ranging from invertebrates such as annelids, cnidarians, echinoderms, molluscs and arthropods to vertebrates such as fish, frogs, snakes, lizards and mammals.
In addition to predation, defence and feeding, we know of at least 11 distinct ecological roles for venom, including its use when competing for mating rights (male platypus) and in the preservation of food (shrews and spider-hunting wasps). Paralysing prey with venom allows it to be stored, alive, for later consumption, or for the laying of eggs and use as a habitat and food source for developing larvae.
Venom production carries a high metabolic cost to the organism, and in all known venomous species the wet weight of venom never exceeds 0.5% of the total body mass of the animal. Replenishing venom raises basal metabolic rates by up to 40%, making it a valuable resource that organisms are reluctant to use on non-prey.
Where possible, venomous organisms display characteristic warning behaviours to alert potential threats to stay away. Rattlesnakes are perhaps the best-known example, creating a rattling noise with their modified tail scalation to alert threats to their location, preventing accidental trampling and identifying that they are dangerous and to be avoided.
Cobras have adopted a more visual defensive behaviour, hooding their necks and raising their head high up off the ground. This behaviour makes the snake as large and intimidating as possible and is often paired with hissing. If these warnings are not heeded, and the perceived threat continues, then the animal will reluctantly bite to protect itself.
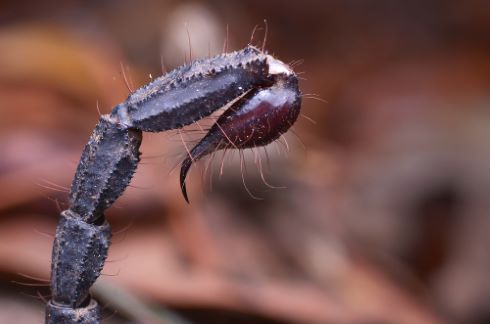
To further save the metabolic cost of producing venom, venomous animals can regulate their venom expenditure, which is known as venom metering. In snakes this is often observed as ‘dry bites’, where the snake bites but no venom is administered. In spiders and scorpions it is more complex – they may take the size of the prey or threat into account, or its movement rate and the risk associated with subduing it, administering venom according to the perceived risk.
Qualitative modulation of venom has also been observed in several species. Parabuthus transvaalicus, a scorpion species from South Africa, has the ability to produce pre-venom and venom. The pre-venom is a clear secretion that causes significant pain and is often injected before the milky protein-rich venom in defensive situations. The pre-venom is likely to have a lower protein content and be less metabolically costly to produce.
The scorpion is able to choose between using dry stings, pre-venom and the metabolically costly milky venom, which is only used in high-threat situations or for predation.
Cone snails and predatory marine snails often possess two distinct venoms – a defensive and a predatory venom, produced in different parts of the venom duct – which it can rapidly switch between. The predatory venom contains prey-specific toxins and has little to no effect on humans. Its defensive venom, however, contains paralytic toxins that can be lethal to humans.
Further research into venom modulation systems is required to better understand the mechanisms at play and to reveal more animals that are capable of modulating their venom secretion.
Venom activity can be divided into three broad categories, depending on their mechanism of activity. Haemotoxic venoms affect the blood, inducing clotting, haemorrhage or cardiovascular distress. Cytotoxic venoms directly affect specific cellular sites and muscles, causing necrosis. And neurotoxic venoms affect the nervous system, disturbing neuron signalling.
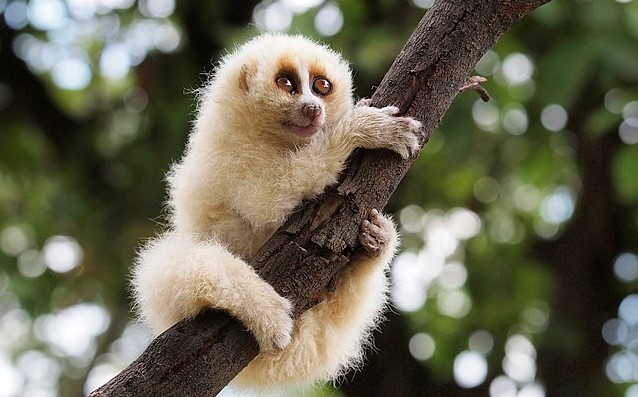
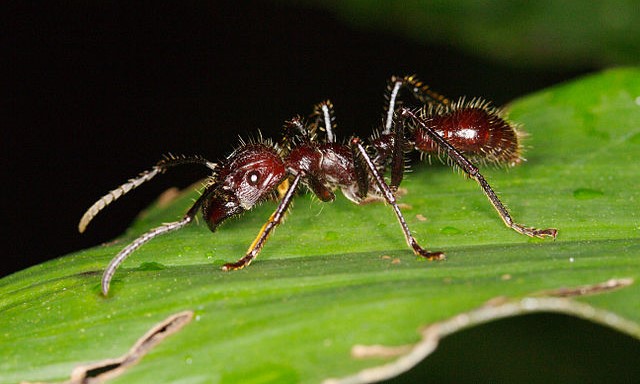
Despite the diversity of venomous animals and their venom composition, there is a high degree of convergent evolution observed in the physiological targets of components of the venom. Convergent targeting across taxa is common and particularly evident in haemostatic and neurological systems, where venom components that have evolved independently into different venomous lineages act on the same molecular targets.
Evolutionary studies have focused primarily on species from younger evolutionary lineages, particularly snakes and cone snails. Venom evolution in ancient lineages, such as cnidarians, coleoids, centipedes and spiders, remains under-studied. Scorpions are the only ancient venomous clade that has received significant research interest. Venom research has also focused almost entirely on the influence of positive selection on venom evolution, ignoring the influence of negative, purifying selection pressures.
These negative selection pressures, which remove mutations that negatively impact the venom toxin efficacy, are essential for the sustained efficacy and potency of venom.
Traditional medicine
Whole venoms have been used in traditional medicines for centuries. The earliest surviving record dates back to 67 BCE, when the venom of the Steppe viper (Vipera ursinii) was used to stop heavy bleeding following a sword wound, saving a patient’s life. In India snake venoms have been used for thousands of years in acupuncture to treat joint pain, and in China the venom from the lumpy brown skin of toads is a traditional anti-cancer treatment.
Venom components work individually or synergistically to target and interfere with physiological and signalling processes, with some venoms containing as many as 1,000 different peptides. Advances in proteomics and ‘venomics’ have enabled the detailed study of these peptides and for peptides to be isolated for use in novel therapeutics, targeting a broad range of conditions including pain, inflammation, cancer, diabetes, cardiovascular diseases and many more.
The first modern commercially available therapeutic derived from venom was developed in the early 1980s. Captopril, an anti-hypotensive medication, was inspired by a peptide isolated from the venom of the Brazilian pit viper (Bothrops jararaca). The peptide acts as an inhibitor to angiotensin-converting enzymes, preventing the release of molecules that cause blood vessel constriction. When taken orally it lowers blood pressure through the relaxation of the circulatory system.
An untapped resource
Since then a further nine venom-derived molecules have been developed into commercially available drugs, with more awaiting approval. Approved medicines include Prialt, a chronic pain medication derived from the powerful venom of cone snails, and Byetta, a type 2 diabetes medication derived from the venom of the Gila monster (a species of venomous lizard).
Some venom-derived compounds have found uses beyond therapeutics that alter physiological processes. Tozuleristide, for example, is a fluorescent biomarker derived from scorpion venom, now used for imaging brain tumours in children. The compound selectively adheres to and illuminates tumours, aiding doctors in differentiating between the tumour and healthy portions of the brain. The compound has completed phase one clinical trials and is currently undergoing phase two testing.
There is a lack of knowledge surrounding the evolutionary and ecological biology of many of the known venomous species, and even less is known about their venom, its composition and mechanism of action. Invertebrates are very poorly studied as a whole, despite them constituting the vast majority of described species on the planet, and this neglect is compounded by the lack of research into their venoms.
Of the 200,000 known venomous species, just a few have been the focus of research into the action or composition of their venoms. Therefore, venoms represent an enormously unexplored natural library of bioactive compounds that have the potential for future drug development.
A more thorough understanding of the evolutionary and ecological biology of a species and its use of venom can then be used to direct bioprospecting efforts, improving the likelihood of identifying and isolating compounds of therapeutic interest.
Thank you to Jarred Williams, a PhD researcher in venom pharmacology at the University of Reading, for his contribution to this article.
Sakthivel Vaiyapuri FRSB is a professor of cardiovascular and venom pharmacology and a Royal Society Leverhulme Trust Senior Research Fellow at the University of Reading.